- Crater Cracks In Welds
- Crater Pipe In Welding Equipment
- Crater Pipe In Welding Supply
- Weld Crater Definition
Welding cracks can be present at the surface, inside of the weld material or at the heat affected zones. Crack can also appear at different temperatures: Hot Crack – It is more prominent during crystallization of weld joints where the temperature can rise more than 10,000-degree Celsius. Craters, such as the one shown in Figure 5A, typically occur at the end of the weld, and they often lead to cracking. Causes include instantly reducing the welding power (which causes the puddle to cool too quickly) and removing the filler rod too quickly at the end of the weld.
Disclaimer: welderportal.com is supported by its audience. When you purchase through links on our site, we may earn a small commission at no extra cost to you.
Defects are common in any type of manufacturing, welding including. In the process, there can be deviations in the shape and size of the metal structure. It can be caused by the use of the incorrect welding process or wrong welding technique. So below we’ll learn about the 7 most common welding defects, their types, causes and remedies.
Table of Contents
- 1 Weld Crack
- 2 Porosity
- 3 Undercut
- 4 Incomplete Fusion
- 5 Incomplete Penetration
- 6 Slag Inclusion
- 7 Spatter
Weld Crack
The most serious type of welding defect is a weld crack and it’s not accepted almost by all standards in the industry. It can appear on the surface, in the weld metal or the area affected by the intense heat.
There are different types of cracks, depending on the temperature at which they occur:
Improper welding technique. Remedies for crater: 1. Using a proper torch angle may reduce the stress on the metal 2. Using a small electrode may also decrease the crater. Use a proper technique. Internal Welding Defects. The various types of internal welding.
- Hot cracks. These can occur during the welding process or during the crystallization process of the weld joint. The temperature at this point can rise over 10,000C.
- Cold cracks. These cracks appear after the weld has been completed and the temperature of the metal has gone down. They can form hours or even days after welding. It mostly happens when welding steel. The cause of this defect is usually deformities in the structure of steel.
- Crater cracks. These occur at the end of the welding process before the operator finishes a pass on the weld joint. They usually form near the end of the weld. When the weld pool cools and solidifies, it needs to have enough volume to overcome shrinkage of the weld metal. Otherwise, it will form a crater crack.
Causes of cracks:
- Use of hydrogen when welding ferrous metals.
- Residual stress caused by the solidification shrinkage.
- Base metal contamination.
- High welding speed but low current.
- No preheat before starting welding.
- Poor joint design.
- A high content of sulfur and carbon in the metal.
Remedies:
- Preheat the metal as required.
- Provide proper cooling of the weld area.
- Use proper joint design.
- Remove impurities.
- Use appropriate metal.
- Make sure to weld a sufficient sectional area.
- Use proper welding speed and amperage current.
- To prevent crater cracks make sure that the crater is properly filled.
Porosity
Porosity occurs as a result of weld metal contamination. The trapped gases create a bubble-filled weld that becomes weak and can with time collapse.
Causes of porosity:
- Inadequate electrode deoxidant.
- Using a longer arc.
- The presence of moisture.
- Improper gas shield.
- Incorrect surface treatment.
- Use of too high gas flow.
- Contaminated surface.
- Presence of rust, paint, grease or oil.
Remedies:
- Clean the materials before you begin welding.
- Use dry electrodes and materials.
- Use correct arc distance.
- Check the gas flow meter and make sure that it’s optimized as required with proper with pressure and flow settings.
- Reduce arc travel speed, which will allow the gases to escape.
- Use the right electrodes.
- Use a proper weld technique.
Crater Cracks In Welds
Undercut
This welding imperfection is the groove formation at the weld toe, reducing the cross-sectional thickness of the base metal. The result is the weakened weld and workpiece.
Causes:
- Too high weld current.
- Too fast weld speed.
- The use of an incorrect angle, which will direct more heat to free edges.
- The electrode is too large.
- Incorrect usage of gas shielding.
- Incorrect filler metal.
- Poor weld technique.
Remedies:
- Use proper electrode angle.
- Reduce the arc length.
- Reduce the electrode’s travel speed, but it also shouldn’t be too slow.
- Choose shielding gas with the correct composition for the material type you’ll be welding.
- Use of proper electrode angle, with more heat directed towards thicker components.
- Use of proper current, reducing it when approaching thinner areas and free edges.
- Choose a correct welding technique that doesn’t involve excessive weaving.
- Use the multipass technique
Incomplete Fusion

This type of welding defect occurs when there’s a lack of proper fusion between the base metal and the weld metal. It can also appear between adjoining weld beads. This creates a gap in the joint that is not filled with molten metal.
Causes:
- Low heat input.
- Surface contamination.
- Electrode angle is incorrect.
- The electrode diameter is incorrect for the material thickness you’re welding.
- Travel speed is too fast.
- The weld pool is too large and it runs ahead of the arc.
Remedies:
- Use a sufficiently high welding current with the appropriate arc voltage.
- Before you begin welding, clean the metal.
- Avoid molten pool from flooding the arc.
- Use correct electrode diameter and angle.
- Reduce deposition rate.
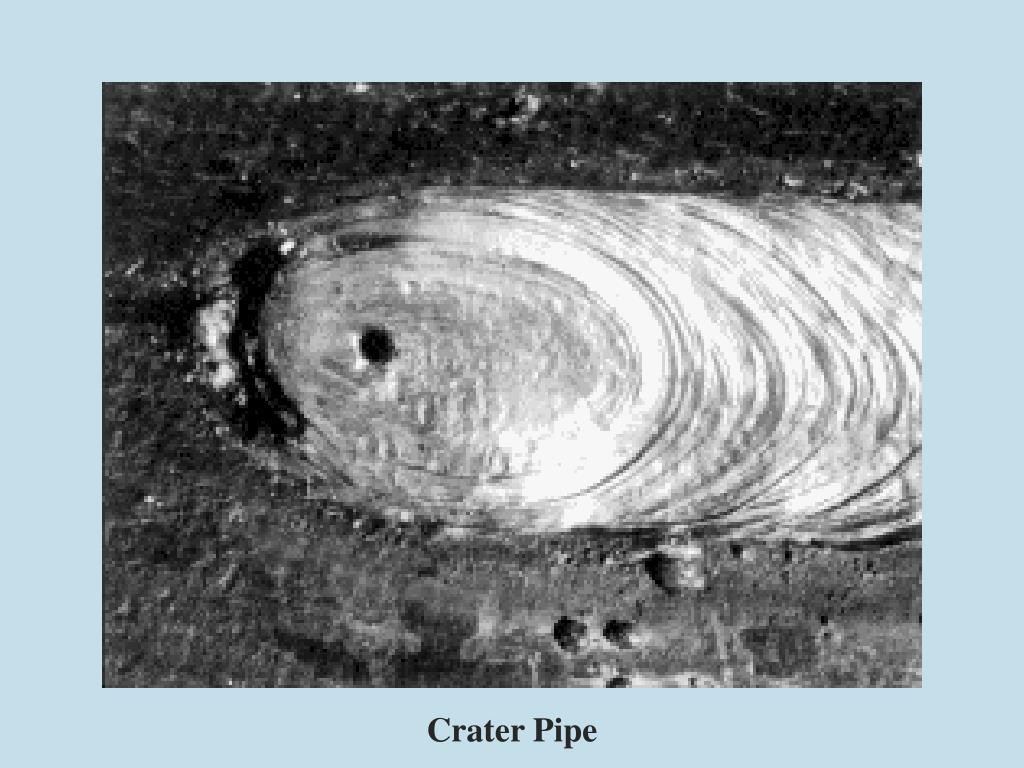
Incomplete Penetration
Incomplete penetration occurs when the groove of the metal is not filled completely, meaning the weld metal doesn’t fully extend through the joint thickness.
Causes:
- There was too much space between the metal you’re welding together.
- You’re moving the bead too quickly, which doesn’t allow enough metal to be deposited in the joint.
- You’re using a too low amperage setting, which results in the current not being strong enough to properly melt the metal.
- Large electrode diameter.
- Misalignment.
- Improper joint.
Remedies:
- Use proper joint geometry.
- Use a properly sized electrode.
- Reduce arc travel speed.
- Choose proper welding current.
- Check for proper alignment.
Slag Inclusion
Slag inclusion is one of the welding defects that are usually easily visible in the weld. Slag is a vitreous material that occurs as a byproduct of stick welding, flux-cored arc welding and submerged arc welding. Is can occur when the flux, which is the solid shielding material used when welding, melts in the weld or on the surface of the weld zone.
Causes:
- Improper cleaning.
- The weld speed is too fast.
- Not cleaning the weld pass before starting a new one.
- Incorrect welding angle.
- The weld pool cools down too fast.
- Welding current is too low.
Remedies:
- Increase current density.
- Reduce rapid cooling.
- Adjust the electrode angle.
- Remove any slag from the previous bead.
- Adjust the welding speed.
Spatter
Spatter occurs when small particles from the weld attach themselves to the surrounding surface. It’s an especially common occurrence in gas metal arc welding. No matter how hard you try, it can’t be completely eliminated. However, there are a few ways you can keep it to a minimum.
Causes:
- The running amperage is too high.
- Voltage setting is too low.
- The work angle of the electrode is too steep.
- The surface is contaminated.
- The arc is too long.
- Incorrect polarity.
- Erratic wire feeding.
Remedies:
- Clean surfaces prior to welding.
- Reduce the arc length.
- Adjust the weld current.
- Increase the electrode angle.
- Use proper polarity.
- Make sure you don’t have any feeding issues.
So we have listed the 7 most common welding defects, their causes and remedies. Upon detecting them, it’s important to remove them in order to prevent the material’s loss of property and strength. Here you can also check out our guide on MIG welding defects troubleshooting.
Related Posts:
The main steps in successful operation of Gas Tungsten Arc Welding (GTAW) include: 1. Electrode Preparation 2. Backing-Up Plates and Purging 3. Arc Initiation 4. Arc Maintenance 5. Current Rectification with A.C. Welding 6. Welding Technique 7. Stopping the Arc.
Step # 1. Electrode Preparation:
Preparing the tungsten electrode properly is very important if a strong, clean, and quality weld is to be achieved. The symmetry of electrode shape determines the gas flow pattern and consequently the degree of protection provided to the molten metal in the weld pool. When the current is loo low or the electrode diameter is too large the arc wanders from point to point particularly when DC EN is used.
This condition can, however, be corrected by grinding the electrode to a point. The electrode tip angle is related to the welding current and the thickness of the material to be welded. It ranges between 30° to 120° but the most common angle used is 60°. The degree of taper also affects the weld penetration; smaller the angle deeper the penetration and narrower the bead.
ADVERTISEMENTS:
The electrode is usually prepared to a balled end with a diameter that must not exceed 1½ times the diameter of the electrode end. Though sometimes the balled end is especially made on an electrode of the shape and size shown in Fig. 9.5 by initially connecting the electrode in the welding circuit with DCEP and the process is interrupted when a ball of the desired size is produced, but in actual use the electrode end assumes the shape depending upon the type of current and polarity, as is shown in Fig. 9.6. The molten hemispherical tip is most desirable for welding.
Thoriated tungsten electrodes do not ball easily and therefore need necessarily to be tapered particularly for welding with low currents. They also give more reliable arc initiation and arc stability with high welding currents.
Electrode protrusion beyond the gas nozzle is determined by the joint design and welding position, for example, in downhand welding the electrode may extend upto 5 mm beyond the nozzle, fillet welds are more difficult to handle from the point of view of access so an extension of upto 6 mm may be desirable, while for corner welds an extension of between 1.5 to 3 mm is adequate. The minimum extension of electrode beyond the nozzle should not be less than 1 .5 mm otherwise the nozzle will get unduly heated up and in all probability be badly damaged.
Step # 2. Backing-Up Plates and Purging:
After GTAW torch is fitted with the properly prepared tungsten electrode but before starting the welding operation it is essential to set the cleaned up work with adequate protection from the backside to avoid any ill effect of the atmospheric gases.
Different methods are used to provide satisfactory backing. One such method is the use of support bars like the carbon black bar commonly employed for the same purpose in oxy-acetylene welding. The second backing method is the introduction of shielding gas on the backside.
This is particularly suited to welding of pipes though it can be used for a plate-like job by providing a support fixture with backing up gas passing through it, as shown in Fig. 9.7. The use of flux backing is another method of protecting the backside of the weld from atmospheric contamination. In case the flux is pasted at the backside it is activated, to produce protective gas, when the temperature goes beyond a particular limit.
For complete protection of backside of the weld it is usually purged with gas flow at the back in a manner similar to the one shown in Fig. 9.7. Gas commonly used for backup is inert gas like argon, however, sometimes nitrogen may be used as purging gas for welding stainless steels. Hydrogen may also be used where the explosion problem is safeguarded against and its absorption by the base metal is not a problem. When it is not possible to provide a supporting fixture for purging or shielding gas then the alternative method is to use oxy-hydrogen flames at the backside. This keeps the underside safe from the atmosphere and its contaminating effects.
Step # 3. Arc Initiation:
Regular flow of electrons is required for arc initiation to take place. Electron emission in tungsten being of the thermionic type, it is imperative to raise the temperature of the electrode tip to start emission of electrons. The ‘touch and draw’ method used for arc initiation in shielded metal arc welding can no doubt be used but it results in the contamination of the tungsten electrode particularly so in the case of high welding current.
This results in lowering of the melting point of the electrode tip which can lead to tungsten inclusion in weld metal, higher consumption of electrode and in the establishment of unstable arc, hence it is an undesirable practice.
In the light of these constraints arc initiation in GTAW is normally done by one of the following three methods:
ADVERTISEMENTS:
(i) Use of carbon block or scrap material,
(ii) High frequency high voltage supply, and
(iii) Low current pilot arc.
It is a common practice to initiate the arc by touch and draw method on a carbon block. The arc is easily established and is sustained for a short while to warm up the tungsten electrode for establishing thermionic emission. This normally takes a few seconds after which the arc is initiated easily at the spot where welding is to be commenced on the workpiece.
This method of touch and draw is not always flawless because carbon Particles may stick to the tungsten electrode which may then be transferred to the workpiece leading to undesirable inclusion or carbon pick up by the weld metal. The tungsten carbide also has a lower melting point and, therefore, results in increasing the size of molten spherical end.
This also results in arc wander and increase in arc resistance which reduces the current density. As these are undesirable conditions it is often recommended to start the arc on scrap of work material till the required warm up of electrode is achieved and then the arc is transferred to the spot where welding is to be commenced.
High voltage high frequency current is often used in conjunction with A.C. power sources to achieve easy arc initiation without touching the electrode to the workpiece. When the high frequency high voltage current is superimposed over the normal welding circuit it quickly ionises the air gap between the electrode tip and the workpiece thereby making it easy for the electron emission to ensue from the tungsten electrode.
The high frequency used ranges between 100 KHz and 2 MHz for a voltage of 3000 to 5000 volts. This method of arc initiation is very efficient and clean and gives a long life to the tungsten electrode. Once the arc is initiated and stabilised the HFHV current is switched off and the normal welding circuit comes into operation. Fig. 9.3 shows the electric circuitry for HFHV arc initiation system and Fig 9.8 depicts the basic waveform obtained with such a unit to initiate or sustain the arc.
Low current pilot arc system is highly reliable arc initiating method which may be used with a d.c. welding system. The pilot arc is established between the tungsten welding electrode and another electrode (usually anode) incorporated in the GTAW torch nozzle, as shown in Fig. 9.9. The pilot arc is powered by a small auxiliary power source and provides conditions for initiating the welding arc in a manner similar to pilot arc used for lighting gas stove. The pilot arc may either be started by scratch technique or by high frequency energy.
Step # 4. Arc Maintenance:
The maintenance of a stable arc is imperative for getting consistent and good quality welds. This may not be much of a problem in a d.c. arc but in a.c. welding the arc voltage and welding current attain zero magnitude every half a cycle. Thus, for a normal 50 Hz mains supply the arc goes off 100 times each second which can lead to arc interruption if adequate measures are not taken to maintain its stability.
This is normally done by one of the following three methods:
(i) High open circuit voltage of the welding transformer,
ADVERTISEMENTS:
(ii) Imposition of high frequency high voltage on the main welding circuit, and
(iii) Injection of high voltage surge.
With the first method a transformer is so designed as to give a sufficiently high OCV and low electrical inertia to help reignite the arc immediately after the current zero pause. During the positive half cycle the electrode runs hotter so there is no need of high OCV on the negative half cycle as the arc re-ignites immediately on change from positive to negative half cycle but at negative to positive half cycle change the electrode is cooler and hence there is delay in re-ignition which results in current zero pause and this phenomenon is known as partial rectification.
During the current zero pause there is jump in voltage to re-ignite the arc as shown in Fig. 9.10. Thus, the arc reignites satisfactorily when the OCV is sufficiently high; this results in a well maintained arc. This method of maintaining a welding arc is also referred to as self re-ignition.
Self re-ignition though simple has its own drawbacks in that the OCV tends to be high, which usually approaches 100 volts and that leads to a low power factor(i.e., Varc/OCV). For greater reliability self re-ignition is often supplemented by providing a high frequency spark unit which is operated from the OCV and becomes inoperative when the voltage drops down to the normal arc operating voltage. This switching off also limits the duration of radio interference.
When HFHV unit is incorporated in the welding circuit for continuous use it can not only be used for arc initiation but also for arc maintenance. To accomplish re-ignition the sparks are discharged across the arc gap and that provides an ionised path for flow of current in the main welding circuit. Slightly lower open circuit voltages are required with high frequency unit and that leads to corresponding improvement in power factor.
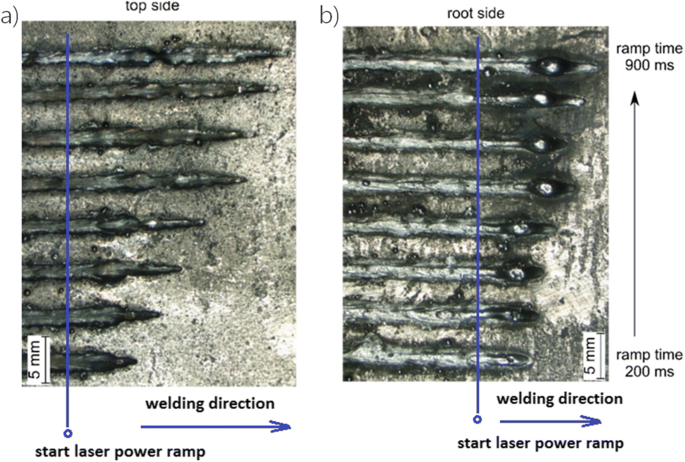
The high frequency spark unit consists of a capacitor that is charged by high voltage transformer which discharges through a spark gap. It is so operated that a train of sparks is generated when the welding power supply voltage exceeds the breakdown voltage of the spark gap and it is arranged to occur during the period when the welding current passes through the current zero pause (see Fig 9.8). It usually covers two-thirds of each half cycle. Due to the cyclic nature of its operation it cannot provide instantaneous arc re-ignition so results in partial rectification.
The third method of arc re-ignition involves injecting a voltage surge into the power circuit to supply the peak voltage required for re-ignition. This is achieved by discharging a capacitor through a switch operated by the power circuit at the intended moment. If the arc goes off at the end of negative half- cycle, the voltage reignition peak starts developing and that fires a gas discharge valve which in turn discharges the capacitor to re-ignite the arc. Re- ignition in this case is instantaneous and thus eliminates the possibility of partial rectification encountered in HFHV method.
As the transformer docs not have to supply the peak OCV therefore the power factor of the system can be improved by utilising a low OCV transformer. The re-ignition infact may be accomplished with 50 volt rms; thus it can also improve the operational safety. The system works momentarily and is automatically switched out once the arc is re-ignited.
The timed voltage surge can only maintain an arc, it cannot initiate it from cold or always after momentary extinction. The circuit diagram for a surge injector and its action are depicted in Fig. 9.11.
Step # 5. Current Rectification with A.C. Welding:
Once a stable arc is established with a. c. in GTAW the tungsten electrode gets heated to a much higher temperature than the temperature of the metal being welded. This results in different abilities of the electrode and the workpiece to emit electrons; the electrode being hotter emits electrons far more readily than the workpiece. This results in difference in resistance to current flow which tends to produce unbalanced a. c. shown in Fig. 9.12.
As higher voltage is needed when the electrode is positive, it results in lower current flow which causes partial rectification. This partial rectification is also known as inherent rectification and results in a d. c component of current which tends to saturate the transformer resulting in its derating to the extent of 30%. This situation is further accentuated due to current zero pauses when they occur.
The detrimental effects of inherent rectification can be corrected by inserting banks of reversible electrolytic capacitors which may give upto 100 pF/A in the power circuit, as shown in Fig. 9.13. This results in a charge being left on these capacitors when the electrode is negative which makes more current to flow when the electrode is positive.
However, the role of this bank of capacitor is reversed at the time of arc initiation when the arc fails during negative cycle of the current. Thus, it results in inverse rectification which leaves a charge of reverse polarity to that for which it is inserted in the circuit. Hence, it opposes the arc initiation. To prevent this the suppressing capacitor is switched out during the period of arc initiation.
When high frequency a.c. is used it is easy to initiate the GTAW arc and if the HF unit is used regularly than arc maintenance is also no problem. In such a case the welding transformer is so designed as to keep the tungsten electrode cool and provide necessary heat balance by modifying the positive and negative half cycles as to give the desired result. For this purpose a positive to a negative half cycle may have a ratio as high as 1: 20, and it may be of any desired configuration, as shown in Fig. 9.14.
Step # 6. Welding Technique:
Both manual and mechanised modes of operation are used for GTAW. For manual welding, after the arc is initiated, the welding torch is held at an angle of 70° to 80° in the forehand welding position. For mechanised GTAW, the welding torch is generally held perpendicular to the workpiece.
To commence manual welding, the arc is moved in a small circle to create a weld pool of suitable size. Once a weld pool of desired size is established at the starting point, a weld is made by moving the torch along the weld joint at the desired welding speed. Solidification of the molten metal gives the desired weld bead shape and the weld is achieved.
Addition or absence of filler metal in GTAW depends upon the work- piece thickness and the joint design. When filler metal is required to be added during manual welding, it is done by hand feeding the filler rod at the leading end of the weld pool.
The welding torch and the filler rod are moved smoothly along the joint edges to maintain a weld pool of consistent size. It is ensured that the shielding gas blanket is maintained over the molten metal until it is solidified and the hot end of the filler rod is also maintained within the shielding gas envelope to avoid the possibility of oxidation.
Different methods of feeding the filler material to the weld pool are adopted. The one most recommended for thin material is that in which the filler rod is held at 15° to the workpiece surface ahead of the torch and it is added repeatedly to the weld pool, as shown in Fig. 9.15. In the second method the filler wire is kept against the workpiece along the weld seam and is melted along with joint edge. For large weld the filler wire is fed continuously into the weld pool; both torch and the filler wire are oscillated but in the opposite direction. In automatic GTAW the filler wire is fed mechanically through a guide into the weld pool at a uniform rate.
From the point of view of good penetration, proper reinforcement, weld quality and economy, downhand or flat welding is best suited for GTAW. However, good penetration can also be achieved in vertical up welding. GTAW torch is usually held at 75° to the workpiece in forehand welding position both for downhand and vertical up welding. Vertical down welding is usually not satisfactory; metal may droop, and lack of penetration often results.
ADVERTISEMENTS:
Mechanised GTAW is frequently employed and often the joints are so designed as to eliminate the need for filler wire. However, when needed the tiller wire of required size is fed into the weld pool from a spool. The mechanised units often make use of arc length control devices, for which the GTAW torch is clamped on a linear actuator and the movement of the torch along it is based on the feedback obtained in terms of change in arc voltage.
This device is very useful to keep consistent arc length and thus can easily eliminate the variation in weld geometry due to minor variation in the workpiece contour. However, it is also employed in automatic GTAW of pipe lines where it automatically adjusts the arc length by shifting the torch position each time a round is completed in a multi-pass circumferential weld. This ensures that the arc voltage and welding current will remain consistent with consequential consistency in weld quality.
Step # 7. Stopping the Arc:
The arc needs to be extinguished at the end of the weld run and this needs to be done gradually rather than abruptly. Sudden stoppage of welding may lead to defects like central pipe and paw cracks. These defects may cause leaks in joints particularly those intended for use in vacuum or under pressure.
The normal method of stopping the arc, therefore, is by reducing the welding speed and withdrawing the torch gradually till the crater is completely filled up. In d.c. welding also the arc is extinguished by lengthening it which leads to increased voltage and reduced current in a manner that depends upon the volt- ampere characteristic of the welding power source.
Crater Pipe In Welding Equipment
In mechanised welding the end crater is reduced by increasing the welding speed before switching off. The crater pipe can also be eliminated by reducing the current gradually before stoppage by employing a device called crater filler.
Crater Pipe In Welding Supply
In all these cases the welding circuit is so designed as to switch on the shielding gas before the flow of current commences in the welding circuit and at the time the torch is switched off the flow of current stops immediately but the shielding gas flow is maintained for a few seconds more to ensure protection of the hot solidifying weld metal. This is achieved by providing solenoid valves in the circuit.
Weld Crater Definition
Related Articles:
